Switch to List View
Image and Video Gallery
This is a searchable collection of scientific photos, illustrations, and videos. The images and videos in this gallery are licensed under Creative Commons Attribution Non-Commercial ShareAlike 3.0. This license lets you remix, tweak, and build upon this work non-commercially, as long as you credit and license your new creations under identical terms.
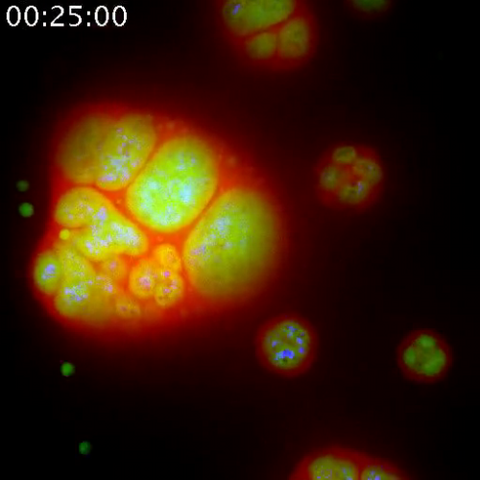
3789: Nucleolus subcompartments spontaneously self-assemble 1
3789: Nucleolus subcompartments spontaneously self-assemble 1
The nucleolus is a small but very important protein complex located in the cell's nucleus. It forms on the chromosomes at the location where the genes for the RNAs are that make up the structure of the ribosome, the indispensable cellular machine that makes proteins from messenger RNAs.
However, how the nucleolus grows and maintains its structure has puzzled scientists for some time. It turns out that even though it looks like a simple liquid blob, it's rather well-organized, consisting of three distinct layers: the fibrillar center, where the RNA polymerase is active; the dense fibrillar component, which is enriched in the protein fibrillarin; and the granular component, which contains a protein called nucleophosmin. Researchers have now discovered that this multilayer structure of the nucleolus arises from difference in how the proteins in each compartment mix with water and with each other. These differences let them readily separate from each other into the three nucleolus compartments.
This video of nucleoli in the eggs of a commonly used lab animal, the frog Xenopus laevis, shows how each of the compartments (the granular component is shown in red, the fibrillarin in yellow-green, and the fibrillar center in blue) spontaneously fuse with each other on encounter without mixing with the other compartments. For more details on this research, see this press release from Princeton. Related to video 3791, image 3792 and image 3793.
However, how the nucleolus grows and maintains its structure has puzzled scientists for some time. It turns out that even though it looks like a simple liquid blob, it's rather well-organized, consisting of three distinct layers: the fibrillar center, where the RNA polymerase is active; the dense fibrillar component, which is enriched in the protein fibrillarin; and the granular component, which contains a protein called nucleophosmin. Researchers have now discovered that this multilayer structure of the nucleolus arises from difference in how the proteins in each compartment mix with water and with each other. These differences let them readily separate from each other into the three nucleolus compartments.
This video of nucleoli in the eggs of a commonly used lab animal, the frog Xenopus laevis, shows how each of the compartments (the granular component is shown in red, the fibrillarin in yellow-green, and the fibrillar center in blue) spontaneously fuse with each other on encounter without mixing with the other compartments. For more details on this research, see this press release from Princeton. Related to video 3791, image 3792 and image 3793.
Nilesh Vaidya, Princeton University
View Media
5760: Annotated TEM cross-section of C. elegans (roundworm)
5760: Annotated TEM cross-section of C. elegans (roundworm)
The worm Caenorhabditis elegans is a popular laboratory animal because its small size and fairly simple body make it easy to study. Scientists use this small worm to answer many research questions in developmental biology, neurobiology, and genetics. This image, which was taken with transmission electron microscopy (TEM), shows a cross-section through C. elegans, revealing various internal structures labeled in the image. You can find a high-resolution image without the annotations at image 5759.
The image is from a figure in an article published in the journal eLife.
The image is from a figure in an article published in the journal eLife.
Piali Sengupta, Brandeis University
View Media

6347: Human Adenovirus
6347: Human Adenovirus
The cryo-EM structure of human adenovirus D26 (HAdV-D26) at near atomic resolution (3.7 Å), determined in collaboration with the NRAMM facility*. In difference to archetype HAdV-C5, the HAdV-D26 is a low seroprevalent viral vector, which is being used to generate Ebola virus vaccines.
National Resource for Automated Molecular Microscopy http://nramm.nysbc.org/nramm-images/ Source: Bridget Carragher
View Media
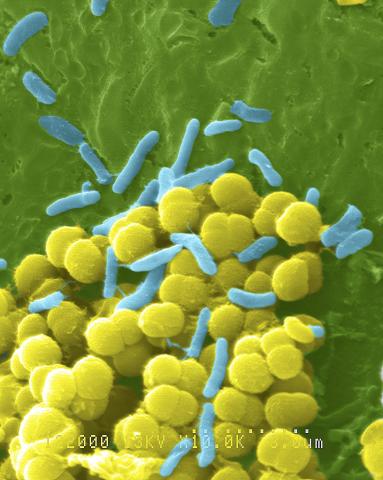
1158: Bacteria shapes
1158: Bacteria shapes
A colorized scanning electron micrograph of bacteria. Scanning electron microscopes allow scientists to see the three-dimensional surface of their samples.
Tina Weatherby Carvalho, University of Hawaii at Manoa
View Media
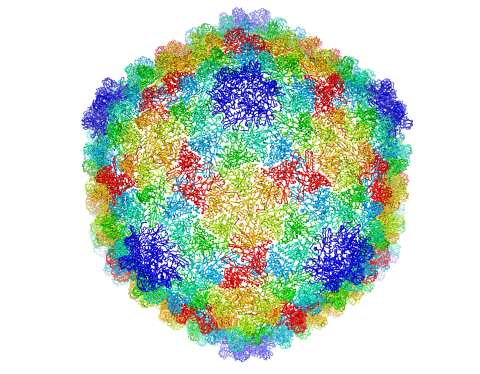
5874: Bacteriophage P22 capsid
5874: Bacteriophage P22 capsid
Cryo-electron microscopy (cryo-EM) has the power to capture details of proteins and other small biological structures at the molecular level. This image shows proteins in the capsid, or outer cover, of bacteriophage P22, a virus that infects the Salmonella bacteria. Each color shows the structure and position of an individual protein in the capsid. Thousands of cryo-EM scans capture the structure and shape of all the individual proteins in the capsid and their position relative to other proteins. A computer model combines these scans into the three-dimension image shown here. Related to image 5875.
Dr. Wah Chiu, Baylor College of Medicine
View Media
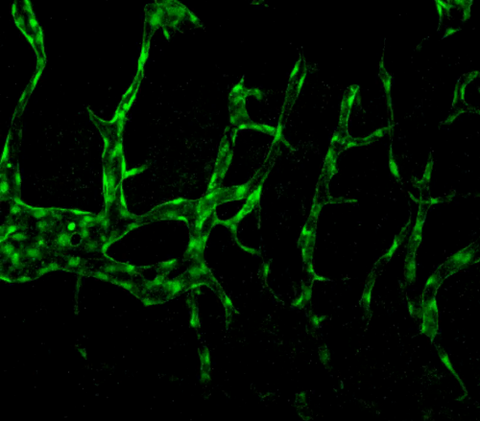
3404: Normal vascular development in frog embryos
3404: Normal vascular development in frog embryos
Hye Ji Cha, University of Texas at Austin
View Media
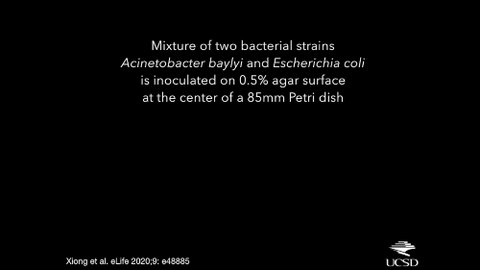
6550: Time-lapse video of floral pattern in a mixture of two bacterial species, Acinetobacter baylyi and Escherichia coli, grown on a semi-solid agar for 24 hours
6550: Time-lapse video of floral pattern in a mixture of two bacterial species, Acinetobacter baylyi and Escherichia coli, grown on a semi-solid agar for 24 hours
This time-lapse video shows the emergence of a flower-like pattern in a mixture of two bacterial species, motile Acinetobacter baylyi and non-motile Escherichia coli (green), that are grown together for 24 hours on 0.75% agar surface from a small inoculum in the center of a Petri dish.
See 6557 for a photo of this process at 24 hours on 0.75% agar surface.
See 6553 for a photo of this process at 48 hours on 1% agar surface.
See 6555 for another photo of this process at 48 hours on 1% agar surface.
See 6556 for a photo of this process at 72 hours on 0.5% agar surface.
See 6557 for a photo of this process at 24 hours on 0.75% agar surface.
See 6553 for a photo of this process at 48 hours on 1% agar surface.
See 6555 for another photo of this process at 48 hours on 1% agar surface.
See 6556 for a photo of this process at 72 hours on 0.5% agar surface.
L. Xiong et al, eLife 2020;9: e48885
View Media
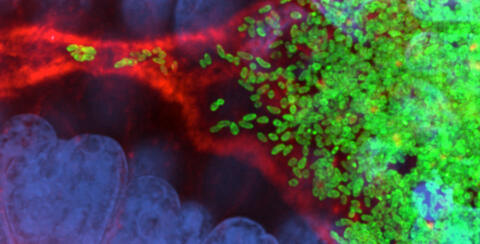
7015: Bacterial cells migrating through the tissues of the squid light organ
7015: Bacterial cells migrating through the tissues of the squid light organ
Vibrio fischeri cells (~ 2 mm), labeled with green fluorescent protein (GFP), passing through a very narrow bottleneck in the tissues (red) of the Hawaiian bobtail squid, Euprymna scolopes, on the way to the crypts where the symbiont population resides. This image was taken using a confocal fluorescence microscope.
Margaret J. McFall-Ngai, Carnegie Institution for Science/California Institute of Technology, and Edward G. Ruby, California Institute of Technology.
View Media
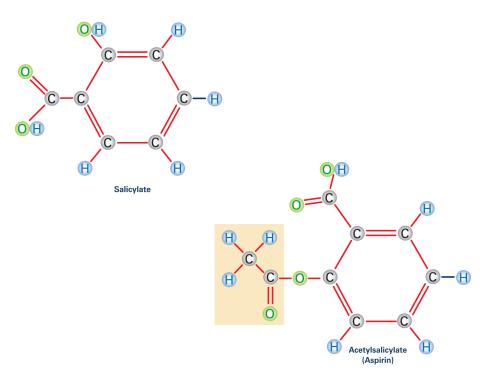
2530: Aspirin (with labels)
2530: Aspirin (with labels)
Acetylsalicylate (bottom) is the aspirin of today. Adding a chemical tag called an acetyl group (shaded box, bottom) to a molecule derived from willow bark (salicylate, top) makes the molecule less acidic (and easier on the lining of the digestive tract), but still effective at relieving pain. See image 2529 for an unlabled version of this illustration. Featured in Medicines By Design.
Crabtree + Company
View Media
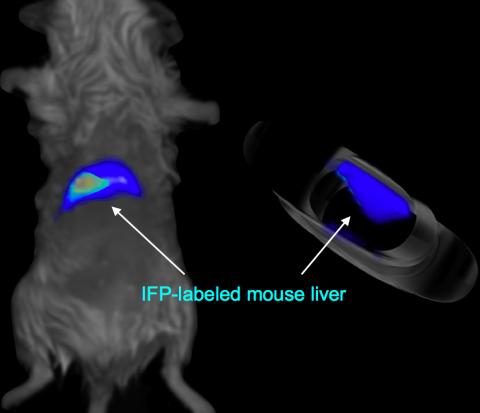
2601: Mouse liver labeled with fluorescent probe
2601: Mouse liver labeled with fluorescent probe
A mouse liver glows after being tagged with specially designed infrared-fluorescent protein (IFP). Since its discovery in 1962, green fluorescent protein (GFP) has become an invaluable resource in biomedical imaging. But because of its short wavelength, the light that makes GFP glow doesn't penetrate far in whole animals. So University of California, San Diego cell biologist Roger Tsien--who shared the 2008 Nobel Prize in chemistry for groundbreaking work with GFP--made infrared-fluorescent proteins (IFPs) that shine under longer-wavelength light, allowing whole-body imaging in small animals.
Xiaokun Shu, University of California, San Diego
View Media
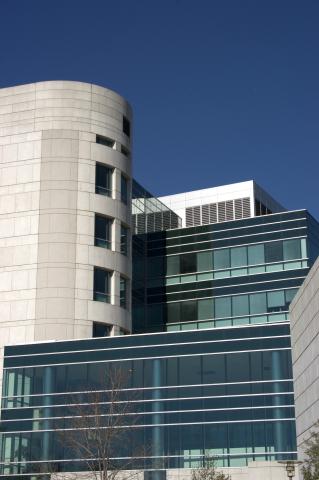
1089: Natcher Building 09
1089: Natcher Building 09
NIGMS staff are located in the Natcher Building on the NIH campus.
Alisa Machalek, National Institute of General Medical Sciences
View Media
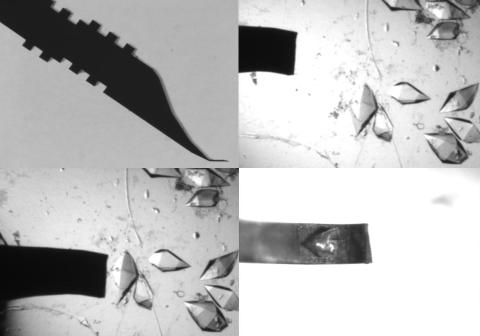
2368: Mounting of protein crystals
2368: Mounting of protein crystals
Automated methods using micromachined silicon are used at the Northeast Collaboratory for Structural Genomics to mount protein crystals for X-ray crystallography.
The Northeast Collaboratory for Structural Genomics
View Media
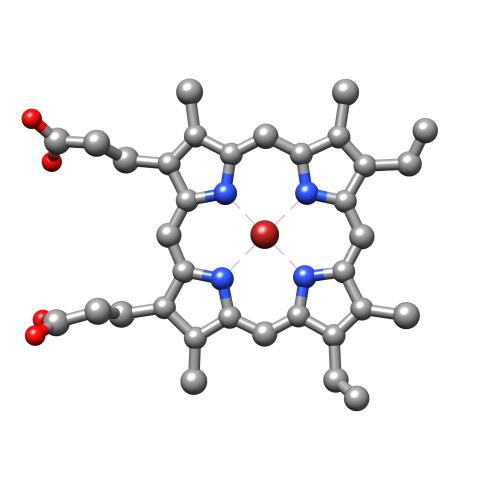
3539: Structure of heme, top view
3539: Structure of heme, top view
Molecular model of the struture of heme. Heme is a small, flat molecule with an iron ion (dark red) at its center. Heme is an essential component of hemoglobin, the protein in blood that carries oxygen throughout our bodies. This image first appeared in the September 2013 issue of Findings Magazine. View side view of heme here 3540.
Rachel Kramer Green, RCSB Protein Data Bank
View Media
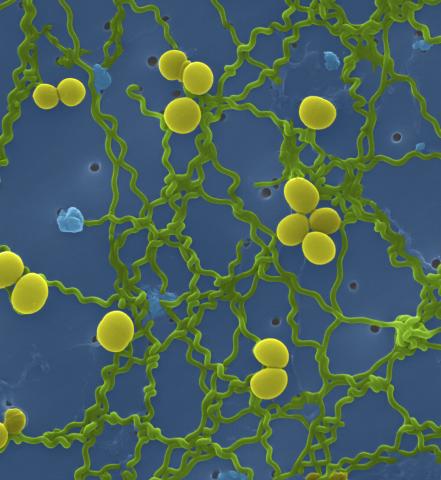
1166: Leptospira bacteria
1166: Leptospira bacteria
Leptospira, shown here in green, is a type (genus) of elongated, spiral-shaped bacteria. Infection can cause Weil's disease, a kind of jaundice, in humans.
Tina Weatherby Carvalho, University of Hawaii at Manoa
View Media
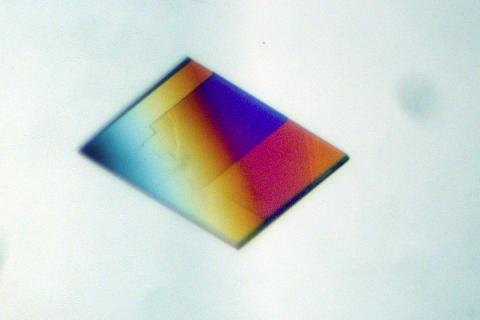
2401: Bacterial alpha amylase
2401: Bacterial alpha amylase
A crystal of bacterial alpha amylase protein created for X-ray crystallography, which can reveal detailed, three-dimensional protein structures.
Alex McPherson, University of California, Irvine
View Media
2434: Fruit fly retina 02
2434: Fruit fly retina 02
Section of a fruit fly retina showing the light-sensing molecules rhodopsin-5 (blue) and rhodopsin-6 (red).
Hermann Steller, Rockefeller University
View Media
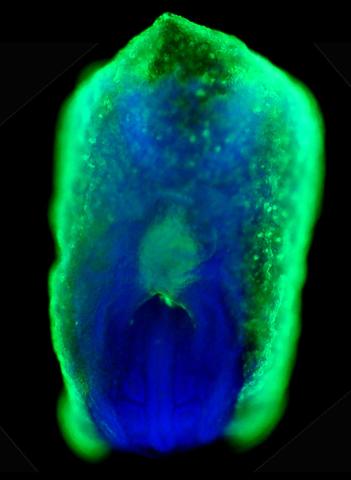
2607: Mouse embryo showing Smad4 protein
2607: Mouse embryo showing Smad4 protein
This eerily glowing blob isn't an alien or a creature from the deep sea--it's a mouse embryo just eight and a half days old. The green shell and core show a protein called Smad4. In the center, Smad4 is telling certain cells to begin forming the mouse's liver and pancreas. Researchers identified a trio of signaling pathways that help switch on Smad4-making genes, starting immature cells on the path to becoming organs. The research could help biologists learn how to grow human liver and pancreas tissue for research, drug testing and regenerative medicine. In addition to NIGMS, NIH's National Institute of Diabetes and Digestive and Kidney Diseases also supported this work.
Kenneth Zaret, Fox Chase Cancer Center
View Media
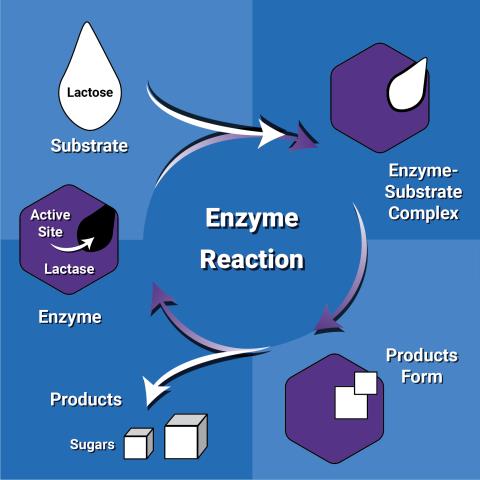
6604: Enzyme reaction
6604: Enzyme reaction
Enzymes speed up chemical reactions by reducing the amount of energy needed for the reactions. The substrate (lactose) binds to the active site of the enzyme (lactase) and is converted into products (sugars).
NIGMS
View Media
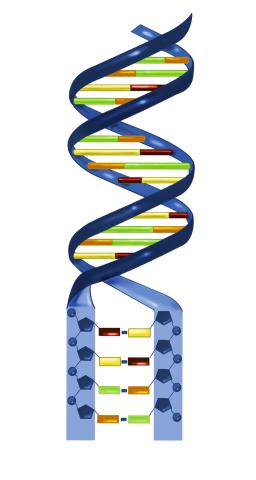
2541: Nucleotides make up DNA
2541: Nucleotides make up DNA
DNA consists of two long, twisted chains made up of nucleotides. Each nucleotide contains one base, one phosphate molecule, and the sugar molecule deoxyribose. The bases in DNA nucleotides are adenine, thymine, cytosine, and guanine. See image 2542 for a labeled version of this illustration. Featured in The New Genetics.
Crabtree + Company
View Media
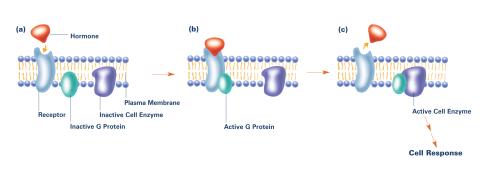
2538: G switch (with labels and stages)
2538: G switch (with labels and stages)
The G switch allows our bodies to respond rapidly to hormones. G proteins act like relay batons to pass messages from circulating hormones into cells. A hormone (red) encounters a receptor (blue) in the membrane of a cell. Next, a G protein (green) becomes activated and makes contact with the receptor to which the hormone is attached. Finally, the G protein passes the hormone's message to the cell by switching on a cell enzyme (purple) that triggers a response. See image 2536 and 2537 for other versions of this image. Featured in Medicines By Design.
Crabtree + Company
View Media
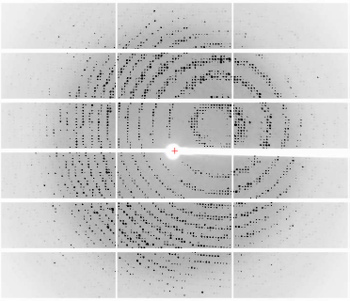
6765: X-ray diffraction pattern from a crystallized cefotaxime-CCD-1 complex
6765: X-ray diffraction pattern from a crystallized cefotaxime-CCD-1 complex
CCD-1 is an enzyme produced by the bacterium Clostridioides difficile that helps it resist antibiotics. Researchers crystallized complexes where a CCD-1 molecule and a molecule of the antibiotic cefotaxime were bound together. Then, they shot X-rays at the complexes to determine their structure—a process known as X-ray crystallography. This image shows the X-ray diffraction pattern of a complex.
Related to images 6764, 6766, and 6767.
Related to images 6764, 6766, and 6767.
Keith Hodgson, Stanford University.
View Media
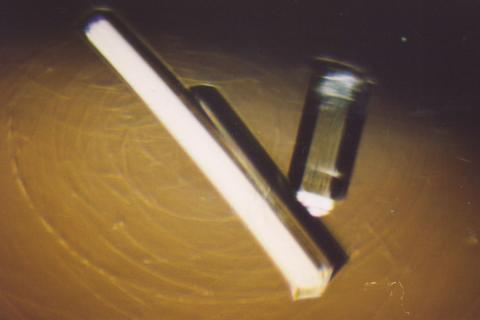
2404: Bovine milk alpha-lactalbumin (2)
2404: Bovine milk alpha-lactalbumin (2)
Crystals of bovine milk alpha-lactalbumin protein created for X-ray crystallography, which can reveal detailed, three-dimensional protein structures.
Alex McPherson, University of California, Irvine
View Media
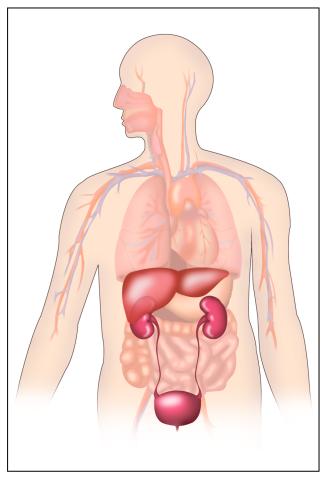
2496: Body toxins
2496: Body toxins
Body organs such as the liver and kidneys process chemicals and toxins. These "target" organs are susceptible to damage caused by these substances. See image 2497 for a labeled version of this illustration.
Crabtree + Company
View Media
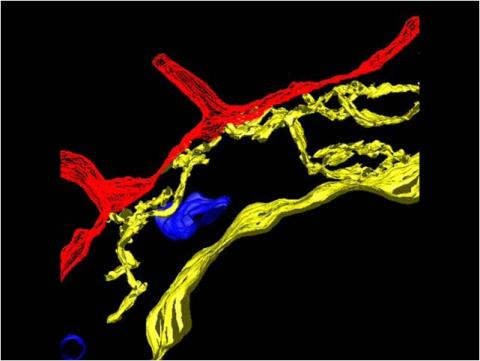
2636: Computer model of cell membrane
2636: Computer model of cell membrane
A computer model of the cell membrane, where the plasma membrane is red, endoplasmic reticulum is yellow, and mitochondria are blue. This image relates to a July 27, 2009 article in Computing Life.
Bridget Wilson, University of New Mexico
View Media
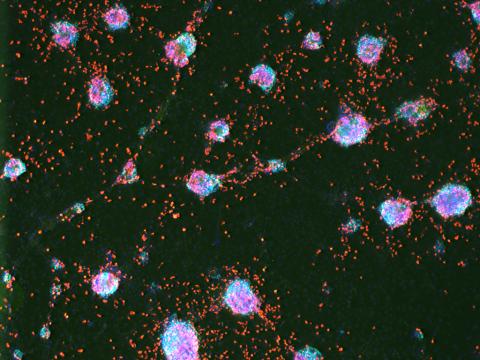
3277: Human ES cells turn into insulin-producing cells
3277: Human ES cells turn into insulin-producing cells
Human embryonic stem cells were differentiated into cells like those found in the pancreas (blue), which give rise to insulin-producing cells (red). When implanted in mice, the stem cell-derived pancreatic cells can replace the insulin that isn't produced in type 1 diabetes. Image and caption information courtesy of the California Institute for Regenerative Medicine.
Eugene Brandon, ViaCyte, via CIRM
View Media
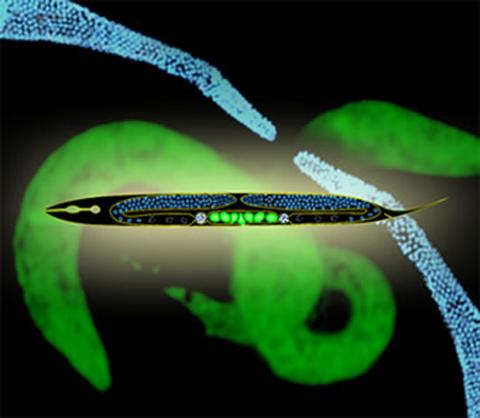
2333: Worms and human infertility
2333: Worms and human infertility
This montage of tiny, transparent C. elegans--or roundworms--may offer insight into understanding human infertility. Researchers used fluorescent dyes to label the worm cells and watch the process of sex cell division, called meiosis, unfold as nuclei (blue) move through the tube-like gonads. Such visualization helps the scientists identify mechanisms that enable these roundworms to reproduce successfully. Because meiosis is similar in all sexually reproducing organisms, what the scientists learn could apply to humans.
Abby Dernburg, Lawrence Berkeley National Laboratory
View Media
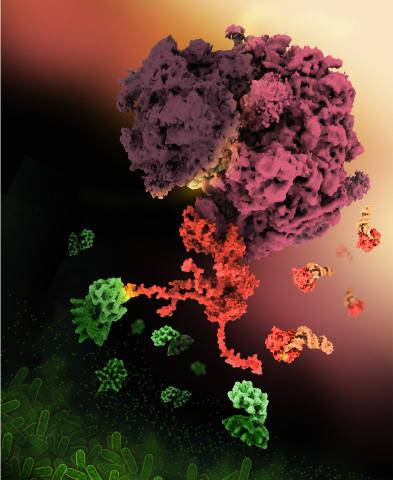
6997: Shiga toxin
6997: Shiga toxin
E. coli bacteria normally live harmlessly in our intestines, but some cause disease by making toxins. One of these toxins, called Shiga toxin (green), inactivates host ribosomes (purple) by mimicking their normal binding partners, the EF-Tu elongation factor (red) complexed with Phe-tRNAPhe (orange).
Find these in the RCSB Protein Data Bank: Shiga toxin 2 (PDB entry 7U6V) and Phe-tRNA (PDB entry 1TTT).
More information about this work can be found in the J. Biol. Chem. paper "Cryo-EM structure of Shiga toxin 2 in complex with the native ribosomal P-stalk reveals residues involved in the binding interaction" by Kulczyk et. al.
Find these in the RCSB Protein Data Bank: Shiga toxin 2 (PDB entry 7U6V) and Phe-tRNA (PDB entry 1TTT).
More information about this work can be found in the J. Biol. Chem. paper "Cryo-EM structure of Shiga toxin 2 in complex with the native ribosomal P-stalk reveals residues involved in the binding interaction" by Kulczyk et. al.
Amy Wu and Christine Zardecki, RCSB Protein Data Bank.
View Media
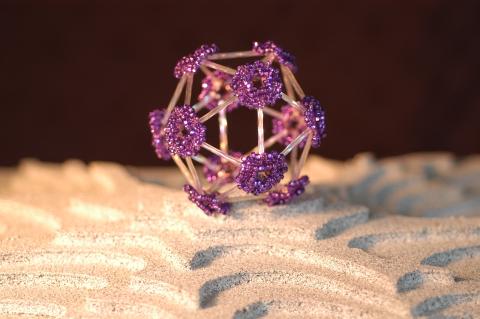
2305: Beaded bacteriophage
2305: Beaded bacteriophage
This sculpture made of purple and clear glass beads depicts bacteriophage Phi174, a virus that infects bacteria. It rests on a surface that portrays an adaptive landscape, a conceptual visualization. The ridges represent the gene combinations associated with the greatest fitness levels of the virus, as measured by how quickly the virus can reproduce itself. Phi174 is an important model system for studies of viral evolution because its genome can readily be sequenced as it evolves under defined laboratory conditions.
Holly Wichman, University of Idaho. (Surface by A. Johnston; photo by J. Palmersheim)
View Media
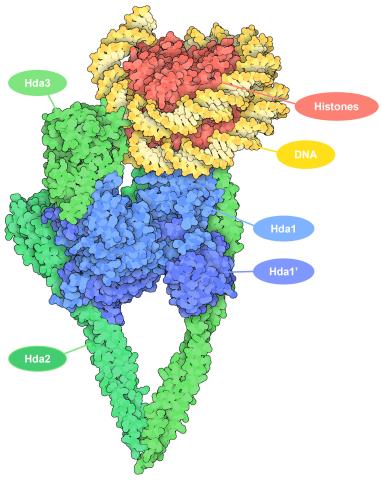
7001: Histone deacetylases
7001: Histone deacetylases
The human genome contains much of the information needed for every cell in the body to function. However, different types of cells often need different types of information. Access to DNA is controlled, in part, by how tightly it’s wrapped around proteins called histones to form nucleosomes. The complex shown here, from yeast cells (PDB entry 6Z6P), includes several histone deacetylase (HDAC) enzymes (green and blue) bound to a nucleosome (histone proteins in red; DNA in yellow). The yeast HDAC enzymes are similar to the human enzymes. Two enzymes form a V-shaped clamp (green) that holds the other others, a dimer of the Hda1 enzymes (blue). In this assembly, Hda1 is activated and positioned to remove acetyl groups from histone tails.
Amy Wu and Christine Zardecki, RCSB Protein Data Bank.
View Media
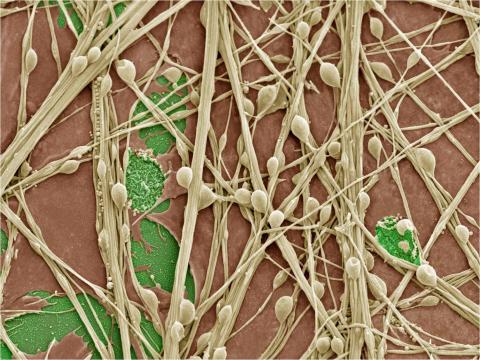
3399: Synapses in culture
3399: Synapses in culture
Cultured hippocampal neurons grown on a substrate of glial cells (astrocytes). The glial cells form the pink/brown underlayment in this image. The tan threads are the neurons. The round tan balls are synapses, the points where neurons meet and communicate with each other. The cover slip underlying the cells is green. Neurons in culture can be used to study synaptic plasticity, activity-dependent protein turnover, and other topics in neuroscience.
National Center for Microscopy and Imaging Research
View Media
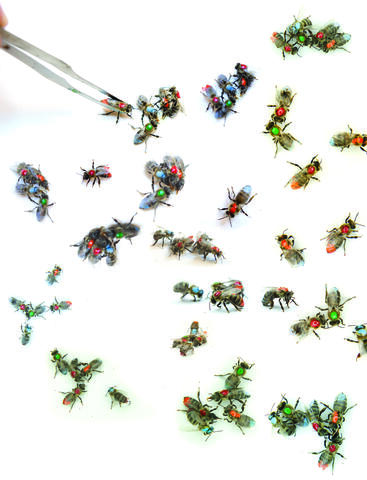
6756: Honeybees marked with paint
6756: Honeybees marked with paint
Researchers doing behavioral experiments with honeybees sometimes use paint or enamel to give individual bees distinguishing marks. The elaborate social structure and impressive learning and navigation abilities of bees make them good models for behavioral and neurobiological research. Since the sequencing of the honeybee genome, published in 2006, bees have been used increasingly for research into the molecular basis for social interaction and other complex behaviors.
Gene Robinson, University of Illinois at Urbana-Champaign.
View Media
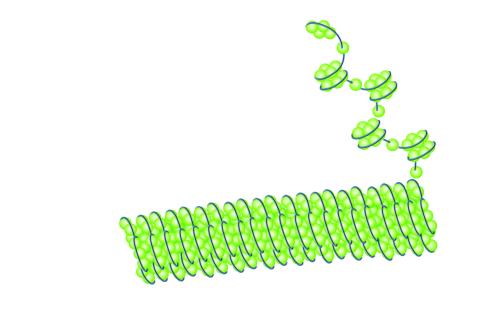
2560: Histones in chromatin
2560: Histones in chromatin
Histone proteins loop together with double-stranded DNA to form a structure that resembles beads on a string. See image 2561 for a labeled version of this illustration. Featured in The New Genetics.
Crabtree + Company
View Media
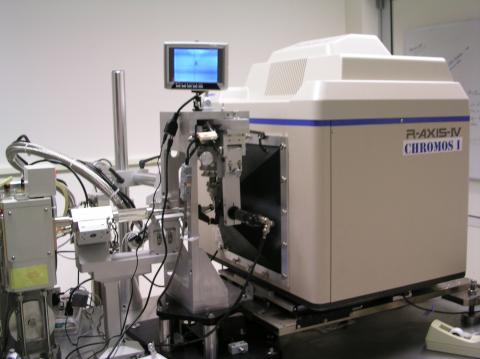
2361: Chromium X-ray source
2361: Chromium X-ray source
In the determination of protein structures by X-ray crystallography, this unique soft (l = 2.29Å) X-ray source is used to collect anomalous scattering data from protein crystals containing light atoms such as sulfur, calcium, zinc and phosphorous. These data can be used to image the protein.
The Southeast Collaboratory for Structural Genomics
View Media
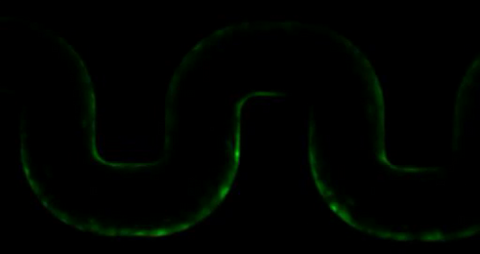
3446: Biofilm blocking fluid flow
3446: Biofilm blocking fluid flow
This time-lapse movie shows that bacterial communities called biofilms can create blockages that prevent fluid flow in devices such as stents and catheters over a period of about 56 hours. This video was featured in a news release from Princeton University.
Bonnie Bassler, Princeton University
View Media
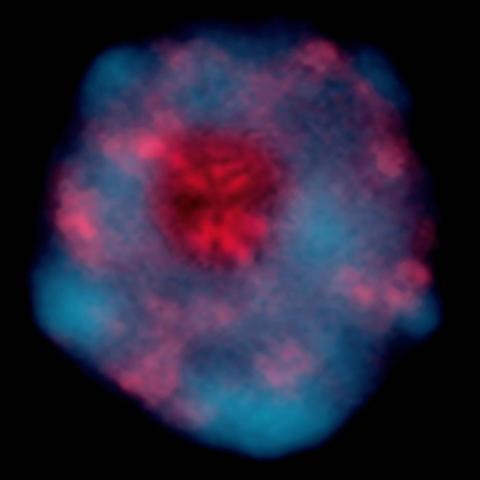
2318: Gene silencing
2318: Gene silencing
Pretty in pink, the enzyme histone deacetylase (HDA6) stands out against a background of blue-tinted DNA in the nucleus of an Arabidopsis plant cell. Here, HDA6 concentrates in the nucleolus (top center), where ribosomal RNA genes reside. The enzyme silences the ribosomal RNA genes from one parent while those from the other parent remain active. This chromosome-specific silencing of ribosomal RNA genes is an unusual phenomenon observed in hybrid plants.
Olga Pontes and Craig Pikaard, Washington University
View Media
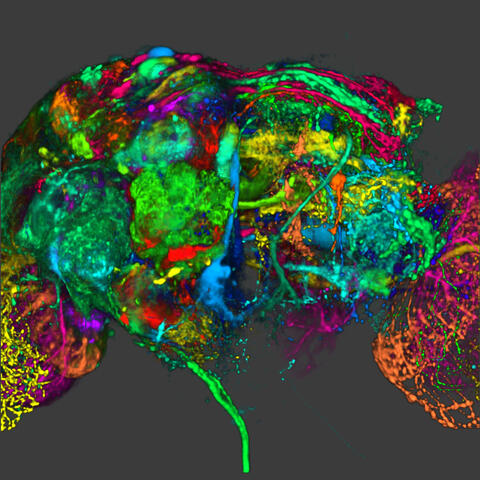
5838: Color coding of the Drosophila brain - image
5838: Color coding of the Drosophila brain - image
This image results from a research project to visualize which regions of the adult fruit fly (Drosophila) brain derive from each neural stem cell. First, researchers collected several thousand fruit fly larvae and fluorescently stained a random stem cell in the brain of each. The idea was to create a population of larvae in which each of the 100 or so neural stem cells was labeled at least once. When the larvae grew to adults, the researchers examined the flies’ brains using confocal microscopy. With this technique, the part of a fly’s brain that derived from a single, labeled stem cell “lights up. The scientists photographed each brain and digitally colorized its lit-up area. By combining thousands of such photos, they created a three-dimensional, color-coded map that shows which part of the Drosophila brain comes from each of its ~100 neural stem cells. In other words, each colored region shows which neurons are the progeny or “clones” of a single stem cell. This work established a hierarchical structure as well as nomenclature for the neurons in the Drosophila brain. Further research will relate functions to structures of the brain.
Related to image 5868 and video 5843
Related to image 5868 and video 5843
Yong Wan from Charles Hansen’s lab, University of Utah. Data preparation and visualization by Masayoshi Ito in the lab of Kei Ito, University of Tokyo.
View Media
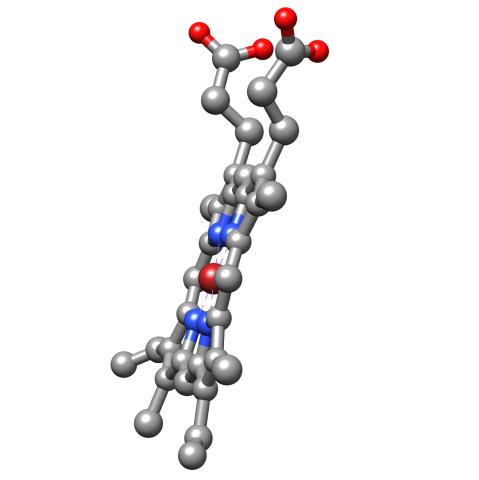
3540: Structure of heme, side view
3540: Structure of heme, side view
Molecular model of the struture of heme. Heme is a small, flat molecule with an iron ion (dark red) at its center. Heme is an essential component of hemoglobin, the protein in blood that carries oxygen throughout our bodies. This image first appeared in the September 2013 issue of Findings Magazine. View side view of heme here 3539.
Rachel Kramer Green, RCSB Protein Data Bank
View Media
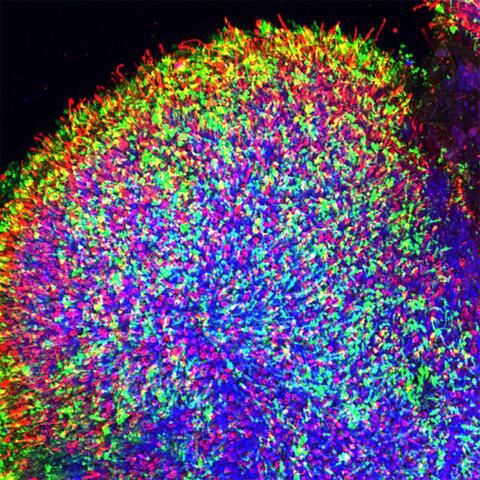
6748: Human retinal organoid
6748: Human retinal organoid
A replica of a human retina grown from stem cells. It shows rod photoreceptors (nerve cells responsible for dark vision) in green and red/green cones (nerve cells responsible for red and green color vision) in red. The cell nuclei are stained blue. This image was captured using a confocal microscope.
Kevin Eliceiri, University of Wisconsin-Madison.
View Media
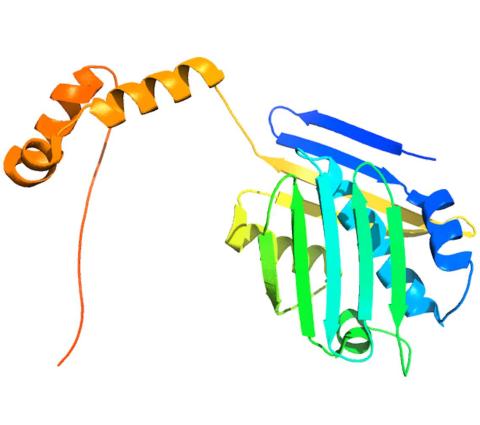
3355: Hsp33 figure 2
3355: Hsp33 figure 2
Featured in the March 15, 2012 issue of Biomedical Beat. Related to Hsp33 Figure 1, image 3354.
Ursula Jakob and Dana Reichmann, University of Michigan
View Media
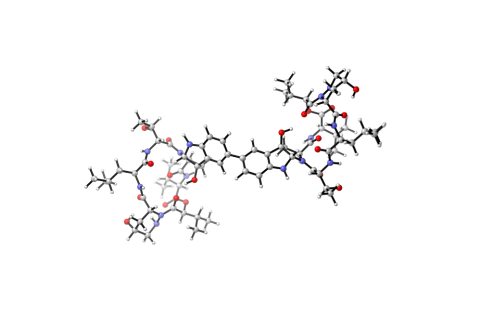
6848: Himastatin
6848: Himastatin
A model of the molecule himastatin, which was first isolated from the bacterium Streptomyces himastatinicus. Himastatin shows antibiotic activity. The researchers who created this image developed a new, more concise way to synthesize himastatin so it can be studied more easily.
More information about the research that produced this image can be found in the Science paper “Total synthesis of himastatin” by D’Angelo et al.
Related to image 6850 and video 6851.
More information about the research that produced this image can be found in the Science paper “Total synthesis of himastatin” by D’Angelo et al.
Related to image 6850 and video 6851.
Mohammad Movassaghi, Massachusetts Institute of Technology.
View Media
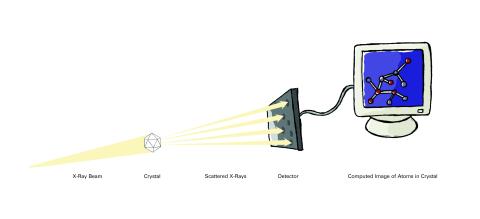
2512: X-ray crystallography (with labels)
2512: X-ray crystallography (with labels)
X-ray crystallography allows researchers to see structures too small to be seen by even the most powerful microscopes. To visualize the arrangement of atoms within molecules, researchers can use the diffraction patterns obtained by passing X-ray beams through crystals of the molecule. This is a common way for solving the structures of proteins. See image 2511 for an unlabeled version of this illustration. Featured in The Structures of Life.
Crabtree + Company
View Media
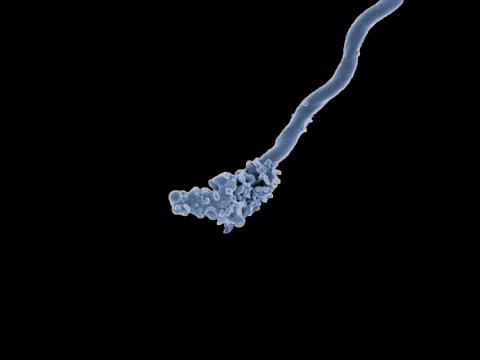
3585: Relapsing fever bacterium (gray) and red blood cells
3585: Relapsing fever bacterium (gray) and red blood cells
Relapsing fever is caused by a bacterium and transmitted by certain soft-bodied ticks or body lice. The disease is seldom fatal in humans, but it can be very serious and prolonged. This scanning electron micrograph shows Borrelia hermsii (green), one of the bacterial species that causes the disease, interacting with red blood cells. Micrograph by Robert Fischer, NIAID. Related to image 3586.
For more information about relapsing fever, see https://www.cdc.gov/relapsing-fever/index.html.
This image is part of the Life: Magnified collection, which was displayed in the Gateway Gallery at Washington Dulles International Airport June 3, 2014, to January 21, 2015.
For more information about relapsing fever, see https://www.cdc.gov/relapsing-fever/index.html.
This image is part of the Life: Magnified collection, which was displayed in the Gateway Gallery at Washington Dulles International Airport June 3, 2014, to January 21, 2015.
NIAID
View Media
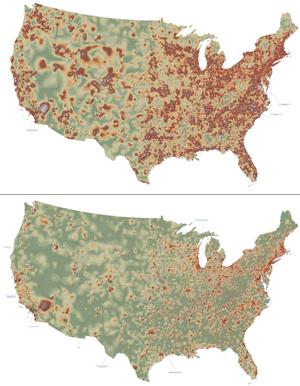
2320: Mapping disease spread
2320: Mapping disease spread
How far and fast an infectious disease spreads across a community depends on many factors, including transportation. These U.S. maps, developed as part of an international study to simulate and analyze disease spread, chart daily commuting patterns. They show where commuters live (top) and where they travel for work (bottom). Green represents the fewest number of people whereas orange, brown, and white depict the most. Such information enables researchers and policymakers to visualize how an outbreak in one area can spread quickly across a geographic region.
David Chrest, RTI International
View Media
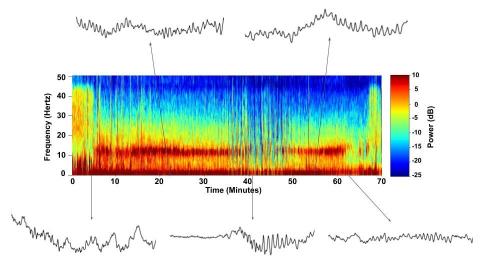
6779: Brain waves of a patient anesthetized with propofol
6779: Brain waves of a patient anesthetized with propofol
A representation of a patient’s brain waves after receiving the anesthetic propofol. All anesthetics create brain wave changes that vary depending on the patient’s age and the type and dose of anesthetic used. These changes are visible in raw electroencephalogram (EEG) readings, but they’re easier to interpret using a spectrogram where the signals are broken down by time (x-axis), frequency (y-axis), and power (color scale). This spectrogram shows the changes in brain waves before, during, and after propofol-induced anesthesia. The patient is unconscious from minute 5, upon propofol administration, through minute 69 (change in power and frequency). But, between minutes 35 and 48, the patient fell into a profound state of unconsciousness (disappearance of dark red oscillations between 8 to 12 Hz), which required the anesthesiologist to adjust the rate of propofol administration. The propofol was stopped at minute 62 and the patient woke up around minute 69.
Emery N. Brown, M.D., Ph.D., Massachusetts General Hospital/Harvard Medical School, Picower Institute for Learning and Memory, and Massachusetts Institute of Technology.
View Media
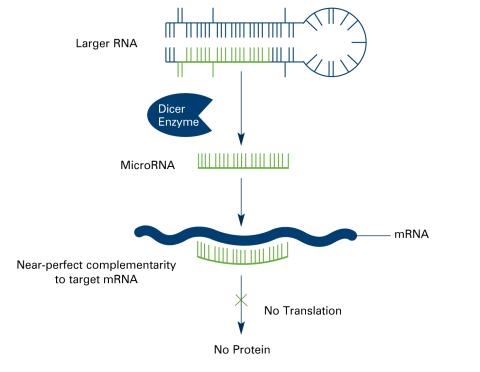
2557: Dicer generates microRNAs (with labels)
2557: Dicer generates microRNAs (with labels)
The enzyme Dicer generates microRNAs by chopping larger RNA molecules into tiny Velcro®-like pieces. MicroRNAs stick to mRNA molecules and prevent the mRNAs from being made into proteins. See image 2556 for an unlabeled version of this illustration. Featured in The New Genetics.
Crabtree + Company
View Media

3567: RSV-Infected Cell
3567: RSV-Infected Cell
Viral RNA (red) in an RSV-infected cell. More information about the research behind this image can be found in a Biomedical Beat Blog posting from January 2014.
Eric Alonas and Philip Santangelo, Georgia Institute of Technology and Emory University
View Media
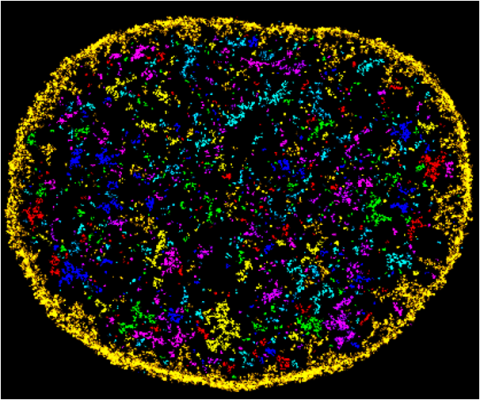
6888: Chromatin in human fibroblast
6888: Chromatin in human fibroblast
The nucleus of a human fibroblast cell with chromatin—a substance made up of DNA and proteins—shown in various colors. Fibroblasts are one of the most common types of cells in mammalian connective tissue, and they play a key role in wound healing and tissue repair. This image was captured using Stochastic Optical Reconstruction Microscopy (STORM).
Related to images 6887 and 6893.
Related to images 6887 and 6893.
Melike Lakadamyali, Perelman School of Medicine at the University of Pennsylvania.
View Media
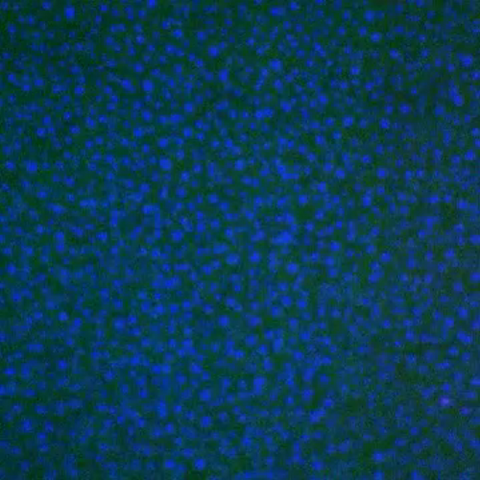
3786: Movie of in vitro assembly of a cell-signaling pathway
3786: Movie of in vitro assembly of a cell-signaling pathway
T cells are white blood cells that are important in defending the body against bacteria, viruses and other pathogens. Each T cell carries proteins, called T-cell receptors, on its surface that are activated when they come in contact with an invader. This activation sets in motion a cascade of biochemical changes inside the T cell to mount a defense against the invasion. Scientists have been interested for some time what happens after a T-cell receptor is activated. One obstacle has been to study how this signaling cascade, or pathway, proceeds inside T cells.
In this video, researchers have created a T-cell receptor pathway consisting of 12 proteins outside the cell on an artificial membrane. The video shows three key steps during the signaling process: phosphorylation of the T-cell receptor (green), clustering of a protein called linker for activation of T cells (LAT) (blue) and polymerization of the cytoskeleton protein actin (red). The findings show that the T-cell receptor signaling proteins self-organize into separate physical and biochemical compartments. This new system of studying molecular pathways outside the cells will enable scientists to better understand how the immune system combats microbes or other agents that cause infection.
To learn more how researchers assembled this T-cell receptor pathway, see this press release from HHMI's Marine Biological Laboratory Whitman Center. Related to image 3787.
In this video, researchers have created a T-cell receptor pathway consisting of 12 proteins outside the cell on an artificial membrane. The video shows three key steps during the signaling process: phosphorylation of the T-cell receptor (green), clustering of a protein called linker for activation of T cells (LAT) (blue) and polymerization of the cytoskeleton protein actin (red). The findings show that the T-cell receptor signaling proteins self-organize into separate physical and biochemical compartments. This new system of studying molecular pathways outside the cells will enable scientists to better understand how the immune system combats microbes or other agents that cause infection.
To learn more how researchers assembled this T-cell receptor pathway, see this press release from HHMI's Marine Biological Laboratory Whitman Center. Related to image 3787.
Xiaolei Su, HHMI Whitman Center of the Marine Biological Laboratory
View Media
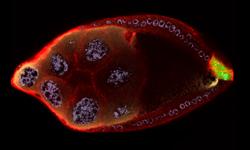
2309: Cellular polarity
2309: Cellular polarity
As an egg cell develops, a process called polarization controls what parts ultimately become the embryo's head and tail. This picture shows an egg of the fruit fly Drosophila. Red and green mark two types of signaling proteins involved in polarization. Disrupting these signals can scramble the body plan of the embryo, leading to severe developmental disorders.
Wu-Min Deng, Florida State University
View Media
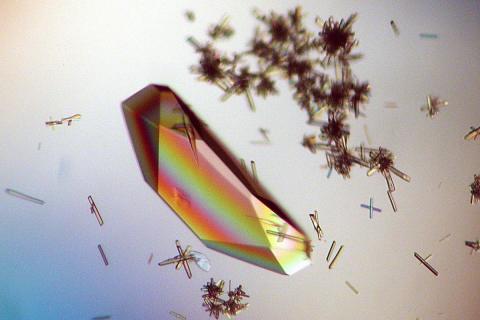
2396: Hen egg lysozyme (1)
2396: Hen egg lysozyme (1)
Crystals of hen egg lysozyme protein created for X-ray crystallography, which can reveal detailed, three-dimensional protein structures.
Alex McPherson, University of California, Irvine
View Media